Using CRISPR To Precisely Kill Dangerous Bacteria
January 2020
In 1928, the first-ever true antibiotic was discovered by Alexander Fleming. Returning from a vacation, he’d accidentally discovered that his Petri dishes had been contaminated by green mold. Now referred to as penicillin notatum, the substance had been killing growing bacteria — and, after further testing, it was found capable of destroying other kinds of bacteria. By preventing bacteria from forming cell walls, they were unable to grow.
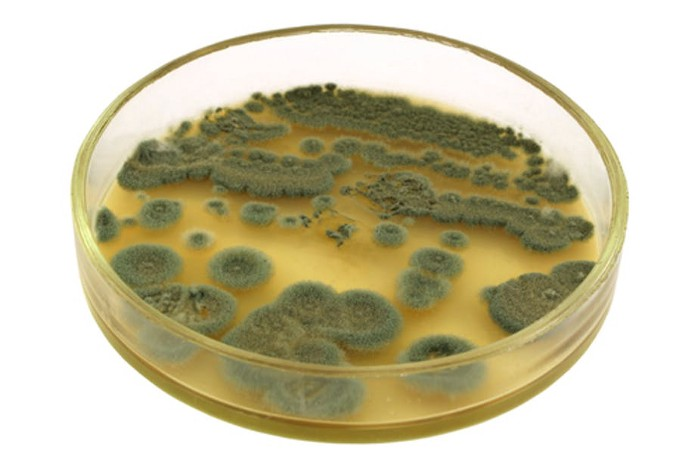
Since then, we’ve come a long way. Antibiotics are now routinely used by people across the planet, with its anti-bacterial benefits helping to keep society healthy. Ciprofloxacin and Cephalexin are just a couple of examples — if you went to your local pharmacy, you’d find tons more.
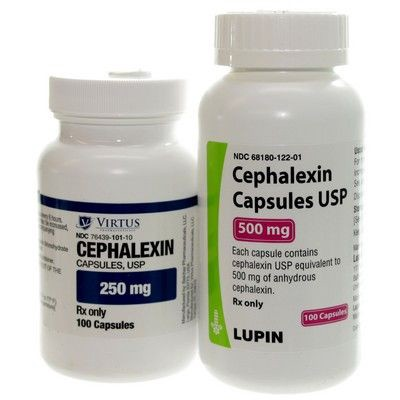
However, our current fight against these single-celled organisms isn’t nearly as perfect as it seems. Resistance to antibiotics is a steadily growing problem, as bacteria like Clostridium difficile, which can cause fatal infections, evades our solutions. Additionally, antibiotics can kill beneficial bacteria instead of solely focusing on targeted organisms.
In fact, in 2015, C. difficile caused nearly half a million infections for Americans — with over 15 000 deaths.
What’s worse is that several more exist — all that have developed resistance to antibiotics, making effective treatment difficult. A list can be found here.
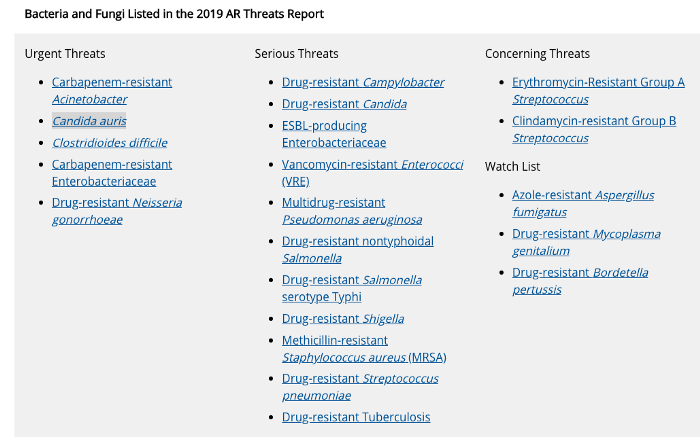
Fortunately, a solution to this issue is being developed! Through leveraging CRISPR as an antibiotic in the form of a pill, scientists are working on specifically killing chosen bacteria.
But what actually is CRISPR?
CRISPR stands for Clustered Regularly Interspaced Short Palindromic Repeats. While this is a mouthful, its meaning is quite simple — repeating sequences of genetic material found in some single-celled organisms. This scientific concept was originally discovered in Escherichia coli, a type of bacteria — more commonly known as E. coli.
To understand what CRISPR’s actual purpose here, think of bacteriophages and E. coli. Bacteriophages are the enemy of bacteria — this virus constantly tries to infect and replicate within bacteria. While harmful to humans, they’re the mortal archnemesis of bacteria. It does this through a tail that pierces a bacterium membrane and injects its genetic material.
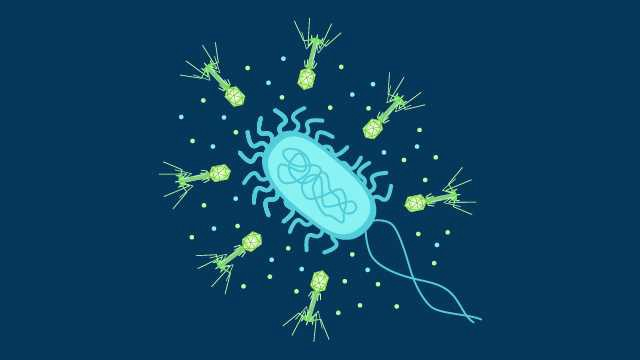
What’s interesting is that CRISPR becomes an immune system of sorts inside of bacteria, effectively fending off bacteriophages.
Within the genome within the bacterium, there are repeating strands of DNA comprised of a palindromic (same phrase backward) sequence of nucleotides. These are our short palindromic repeats.
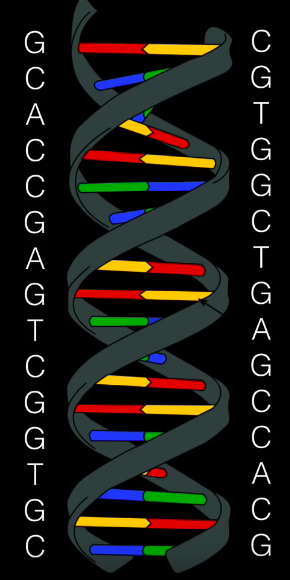
However, there are spaces between these repeats of DNA within the bacterium’s genetic sequence. These are filled with spacer DNA, the material that’s important. Additionally, each segment of this type of DNA is different.

And thus we have our ‘Clustered Regularly Interspaced Short Palindromic Repeats’ — our spacer DNA segments are what make our palindromic repeats ‘interspaced’.
These spacer DNA genes match up with bacteriophage DNA. Further genes exist in a bacterium’s genome that are CRISPR-associated and are therefore called cas genes. These cas genes make Cas proteins — which are helicases and nucleases. This means that these proteins have the capability to unzip and splice genetic material.
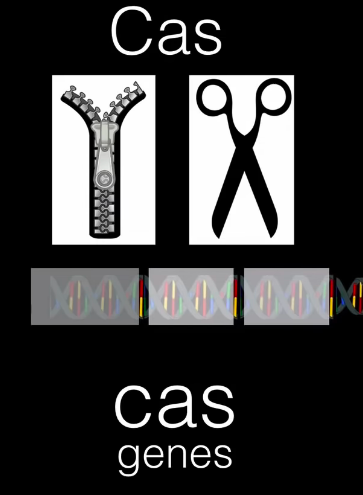
CRISPR DNA and Cas proteins come together now to combat bacteriophages!
When bacteriophages inject their DNA into the bacterium, the spacer DNA segment that is identical to the injected DNA is transcribed to make CRISPR RNA (crRNA). In addition, the Cas protein will be made from the cas genes. The crRNA and Cas come together to destroy the inserted piece of DNA.
If foreign DNA is inserted from a bacteriophage, when there isn’t a corresponding segment DNA, the Cas protein destroys it, and copies it, and adds it to the sequence.
Using the Cas9 Protein to Splice DNA — The CRISPR Cas9 System
The specific Cas protein, Cas9, works with crRNA and tracrRNA. The crRNA binds to the target DNA strand so it can be spliced, and the tracrRNA essentially holds the crRNA in place. Both of these RNA strands are commonly referred to combined as gRNA — the guide RNA for the Cas9 protein, specifying and binding to the targeted piece of DNA.
In short, CRISPR Cas9 comprises of the Cas9 protein and the gRNA.
Here’s an analogy to understand how CRISPR Cas9 functions. Imagine you’re in the woods, shooting beer cans with a rifle.
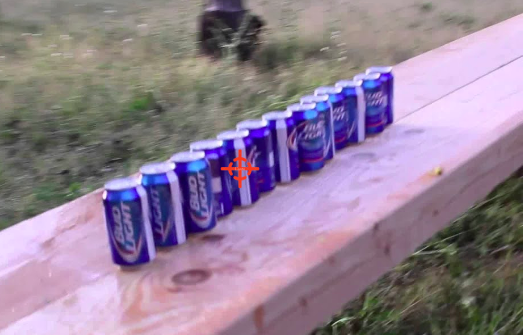
The crosshair is the guide RNA — specifying our target and locking onto it. Our gun is the Cas9 protein — actually doing the damage to our target.
Let’s discuss what this would look like with DNA
Firstly, we make a gRNA so that it can bond with our target DNA sequence. This is how we specify our Cas9 protein where to splice precisely. Guanine (G) always pairs with cytosine (C), and adenine (A) always pairs with thymine (T). So, if our target segment had the code ATCG we’d make a gRNA with the code TAGC.
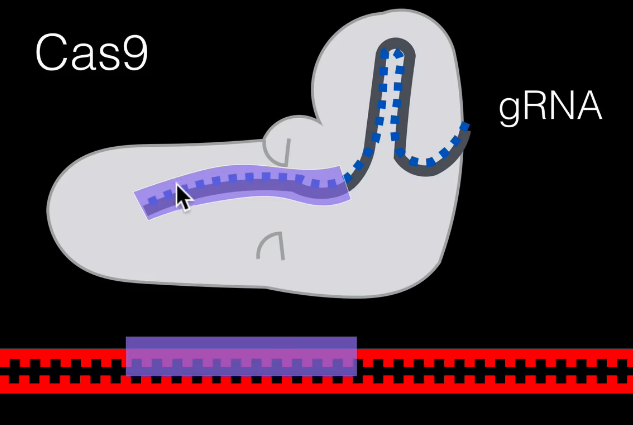
Because our Cas9 protein is a helicase, it unzips our DNA strand. Eventually, our guide RNA will pair with the intended sequence, specifying where the strand needs to be cleaved.
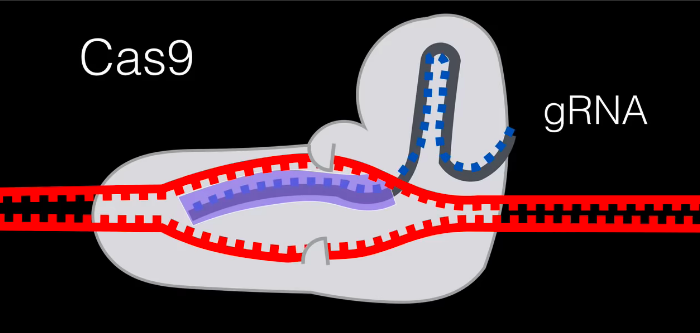
The ‘pincers’ in this diagram are where the protein’s nuclease functions come out. They’re in charge of splicing the DNA.
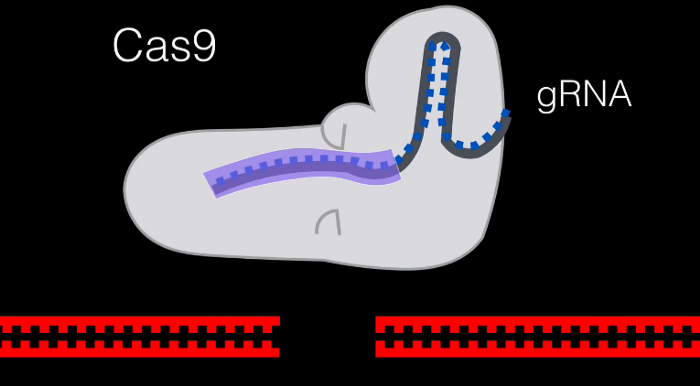
We now have an inactive gene. The cell can add or delete DNA to try and repair and mutate the gene naturally. If we wanted to choose what the gene should look like, we can create a custom host RNA to be added.
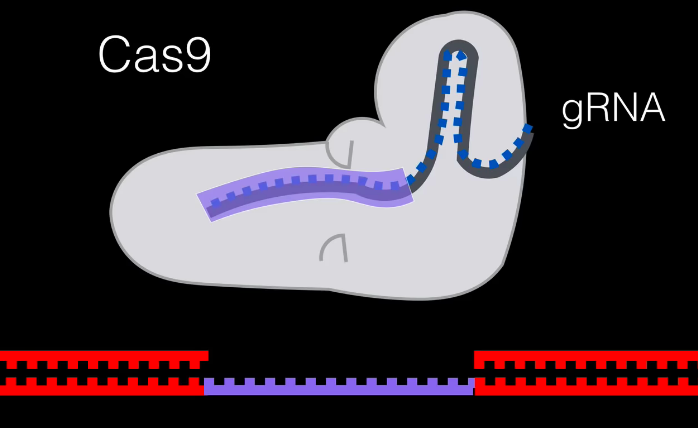
Our cell will repair the rest of the DNA manually, and therefore our gene has been modified to our liking.
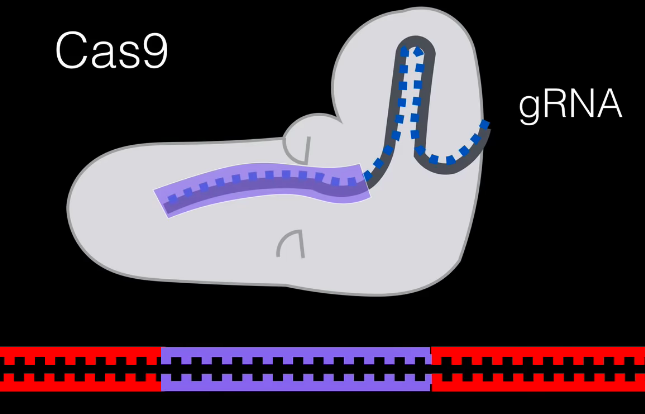
This entire process is how humans have the potential change genes — technically referred to as gene editing.
Alright, that was a lot of information. You’re probably thinking — how the heck are scientists using this to tackle antibiotic resistance?
Using CRISPR to Specifically Kill Bacteria of Choice
What Jan-Peter Van Pjikeren’s looking into is scaling CRISPR technology to a new domain — an “ultra-precise microbial treatment” so that it can kill the bacteria of choice. Not only does this overcome the growing issues of resistance for conventional antibiotics, but it also ensures that beneficial bacteria aren’t harmed — only negative ones that're intended for destruction. For example, C. difficile.
How would this work?
The central idea is somewhat similar to the origins of CRISPR — bacteria, and bacteriophages. With a bacteriophage, the “wrong message” can be sent to targeted bacteria so that it ends up splicing its own DNA, effectively killing itself. By sending a false signal, in theory, the bacterium’s Cas9 protein would cleave its own DNA.
If Cas9 didn’t have bacteriophage DNA to splice, it’d end up destroying the identical spacer DNA segment instead. When the bacterium’s strand of genetic material is cut, it’s essentially committed suicide.
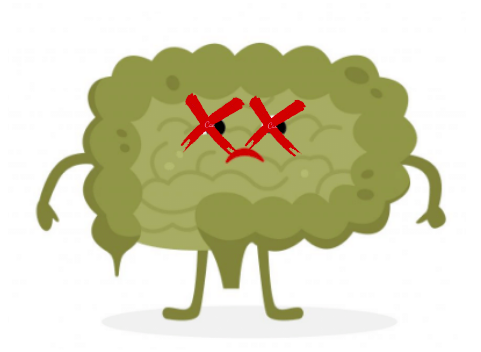
However, bacteriophages on their own would get broken down by stomach acids before significantly combatting target bacteria like C. difficile. This is why scientists are coating it with harmless bacteria so that it takes the form of a pill or a liquid. In a sense, this transportation system for our bacteriophage is a “probiotic mothership”!
As the mothership moves through the intestinal tract, once C. difficile bacteria are near, the bacteriophage bursts forward and inserts its false signal into the bacterium. This causes the latter to mess up its own DNA and self-destruct.
The Future of Antibiotics
While this idea is still coming to fruition, imagine its benefits! We’d be able to specifically and precisely tackle deadly bacteria in the human body, and overcome the issue of antibiotic resistance. By using probiotic motherships and bacteriophages, we can replace ordinary antibiotics!
With traditional antibiotics, tackling areas like the gut tend to wipe out tons of helpful organisms. CRISPR + bacteriophages = specificity.
Through the use of CRISPR, we’re literally able to cause hazardous bacteria to self-destruct through its gene modifying properties. Our first way of tackling bacteria was found accidentally nearly a century ago — now, we’re finding ways to precisely hack organisms. What more will we see in the future?
Key Takeaways
- Antibiotic resistance is a growing issue in America.
- Traditional antibiotics have downsides, including affecting beneficial bacteria
- Tons of dangerous bacteria are finding ways to evade antibiotic treatment, including Clostridium difficile, which infected over half a million Americans in 2015.
- CRISPR (Clustered Regularly Interspaced Short Palindromic Repeats) Cas9 is a system for the splicing and editing of DNA sequences.
- The process for modifying DNA with CRISPR uses guide RNA and the Cas9 protein.
- Using CRISPR with bacteriophages, scientists are working on tricking harmful bacteria to self-destruct by ruining their own genome.
- This could be the future of anti-bacterial treatment!
Further Learning
I got some of my information and media from these sources! I suggest you check them out: